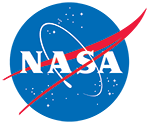
GLAS/ICESat L2 Global Land Surface Altimetry Data, Version 34
Data set id:
GLA14
DOI: 10.5067/ICESAT/GLAS/DATA227
This data set has been retired.
Version Summary
Version Summary
Release 34 incorporates fixes for several data issues that were determined to exist in the GLAS Release 33 data products.
Overview
NOTE ON GLAS BINARY DATA: Access to all ICESat/GLAS binary data products at NSIDC DAAC was removed 01 August 2017. The Binary Data Subsetter also has been decommissioned. ICESat/GLAS data remain available in HDF5 format.
The Geoscience Laser Altimeter System (GLAS) instrument on the Ice, Cloud, and Land Elevation Satellite (ICESat) provides global measurements of polar ice sheet elevation to discern changes in ice volume (mass balance) over time. Secondary objectives of GLAS are to measure sea ice roughness and thickness, cloud and atmospheric properties, land topography, vegetation canopy heights, ocean surface topography, and surface reflectivity.
GLAS has a 1064 nm laser channel for surface altimetry and dense cloud heights, and a 532 nm lidar channel for the vertical distribution of clouds and aerosols.
This level-2 altimetry product (GLA14) provides surface elevations for land. Data also include the laser footprint gelocation and reflectance, as well as geodetic, instrument, and atmospheric corrections for range measurements.
Each data granule has an associated browse product that users can quickly view to determine the general quality of the data in the granule. Browse products consist of image plots of key parameters and statistics. Data are in scaled integer binary format, big-endian (Unix) byte order.
Parameter(s):
LASER REFLECTANCETERRAIN ELEVATION
Platform(s):
ICESAT
Sensor(s):
ALTIMETERS, GLAS
Data Format(s):
Binary
Temporal Coverage:
20 February 2003 to 11 October 2009
Temporal Resolution:
- 1288 minute
Spatial Resolution:
- 60 m to 70 m
- 60 m to 70 m
Spatial Coverage:
N:
86
S:
-86
E:
180
W:
-180
Blue outlined yellow areas on the map below indicate the spatial coverage for this data set.
Data Access & Tools
Documentation
User Guide
General Resources
Product Specification Documents
Help Articles
General Questions & FAQs
The ICESat/GLAS elevation data are relative to the ellipsoid.
The data also have a parameter indicating the geoid height, which is the height of the EGM2008 geoid above the TOPEX/Poseidon ellipsoid for the first and last shot in the record:
How to Articles
Many NSIDC DAAC data sets can be accessed using the NSIDC DAAC's Data Access Tool. This tool provides the ability to search and filter data with spatial and temporal constraints using a map-based interface.Users have the option to
Harmony API Quickstart Guide: Customizing NASA NSIDC DAAC data in Earthdata Cloud