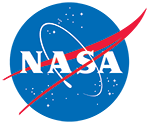
IceBridge MCoRDS L1B Geolocated Radar Echo Strength Profiles, Version 2
Data set id:
IRMCR1B
DOI: 10.5067/90S1XZRBAX5N
This is the most recent version of these data.
Version Summary
Version Summary
Version 2 data are in netCDF format beginning with the 2012 Antarctica campaign.
Version 1 data are in MATLAB and binary format for Spring 2012 and earlier campaigns.
Version 1 data are in MATLAB and binary format for Spring 2012 and earlier campaigns.
- Beginning with the 2012 Antarctica campaign, the data file format is netCDF.
- Data files for all previous campaigns are to be replaced with netCDF files.
Overview
This data set contains radar echograms taken from the Center for Remote Sensing of Ice Sheets (CReSIS) ultra Multichannel Coherent Radar Depth Sounder (MCoRDS) over land and sea ice in the Arctic and Antarctic.
Parameter(s):
RADAR IMAGERYSEA ICE ELEVATION
Platform(s):
BT-67, C-130, DC-8, G-V, NASA DC-8, NOAA Twin Otter, P-3B, WP-3D ORION
Sensor(s):
MCoRDS
Data Format(s):
NetCDF, JPEG
Temporal Coverage:
16 October 2009 to 20 November 2019
Temporal Resolution:
- Varies
Spatial Resolution:
- varies
- varies
Spatial Coverage:
N:
83.1
S:
59
E:
28.5
W:
-100.2
N:
-57
S:
-90
E:
180
W:
-180
Blue outlined yellow areas on the map below indicate the spatial coverage for this data set.
Data Access & Tools
A free NASA Earthdata Login account is required to access these data. Learn More
Documentation
Help Articles
How to Articles
Many NSIDC DAAC data sets can be accessed using the NSIDC DAAC's Data Access Tool. This tool provides the ability to search and filter data with spatial and temporal constraints using a map-based interface.Users have the option to
Data from the NASA National Snow and Ice Data Center Distributed Active Archive Center (NSIDC DAAC) can be accessed directly from our HTTPS file system. We provide basic command line Wget and curl instructions for HTTPS file system downloads below.
All data from the NASA National Snow and Ice Data Center Distributed Active Archive Center (NSIDC DAAC) is directly accessible through our HTTPS file system using Wget or curl. This article provides basic command line instructions for accessing data using this method.