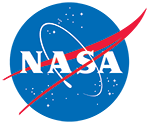
IceBridge ATM L1B Elevation and Return Strength with Waveforms, Version 1
Data set id:
ILATMW1B
DOI: 10.5067/EZQ5U3R3XWBS
This is the most recent version of these data.
Version Summary
Version Summary
Initial release
Overview
This data set contains spot elevation measurements with corresponding waveforms of Arctic and Antarctic sea ice, and Greenland, Antarctic Peninsula, and West Antarctic region ice surface acquired using the NASA Airborne Topographic Mapper (ATM) instrumentation. The data were collected as part of Operation IceBridge funded aircraft survey campaigns.
Parameter(s):
GLACIER ELEVATION/ICE SHEET ELEVATIONSENSOR COUNTS
Platform(s):
C-130, DC-8, G-V, HU-25A, HU-25C, P-3B, WP-3D ORION
Sensor(s):
ATM
Data Format(s):
HDF5
Temporal Coverage:
17 July 2017 to 20 November 2019
Temporal Resolution:
- Varies
Spatial Resolution:
- varies
- varies
Spatial Coverage:
N:
90
S:
60
E:
180
W:
-180
N:
-53
S:
-90
E:
180
W:
-180
Blue outlined yellow areas on the map below indicate the spatial coverage for this data set.
Data Access & Tools
A free NASA Earthdata Login account is required to access these data. Learn More
Documentation
Help Articles
How to Articles
Many NSIDC DAAC data sets can be accessed using the NSIDC DAAC's Data Access Tool. This tool provides the ability to search and filter data with spatial and temporal constraints using a map-based interface.Users have the option to
To convert HDF5 files into binary format you will need to use the h5dump utility, which is part of the HDF5 distribution available from the HDF Group. How you install HDF5 depends on your operating system.
All data from the NASA National Snow and Ice Data Center Distributed Active Archive Center (NSIDC DAAC) can be accessed directly from our HTTPS file system, using wget or curl. Basic command line instructions are provided in the article below.
NASA Earthdata Search is a map-based interface where a user can search for Earth science data, filter results based on spatial and temporal constraints, and order data with customizations including re-formatting, re-projecting, and spatial and parameter subsetting.