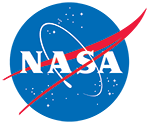
GLAS/ICESat L2 Global Cloud Heights for Multi-layer Clouds, Version 33
Data set id:
GLA09
DOI: 10.5067/ICESAT/GLAS/DATA222
This data set has been retired.
Version Summary
Version Summary
For information about Version 33, see the NSIDC ICESat Description of Data Releases page.
Overview
NOTE ON GLAS BINARY DATA: Access to all ICESat/GLAS binary data products at NSIDC DAAC was removed 01 August 2017. The Binary Data Subsetter also has been decommissioned. ICESat/GLAS data remain available in HDF5 format.
The Geoscience Laser Altimeter System (GLAS) instrument on the Ice, Cloud, and Land Elevation Satellite (ICESat) provides global measurements of polar ice sheet elevation to discern changes in ice volume (mass balance) over time. Secondary objectives of GLAS are to measure sea ice roughness and thickness, cloud and atmospheric properties, land topography, vegetation canopy heights, ocean surface topography, and surface reflectivity.
GLAS has a 1064 nm laser channel for surface altimetry and dense cloud heights, and a 532 nm lidar channel for the vertical distribution of clouds and aerosols.
Level-2 cloud heights for multi-layer clouds (GLA09) contain cloud layer top and bottom height data at sampling rates of 4 sec, 1 sec, 5 Hz, and 40 Hz.
Each data granule has an associated browse product that users can quickly view to determine the general quality of the data in the granule. Browse products consist of image plots of key parameters and statistics. Data are in scaled integer binary format, big-endian (Unix) byte order.
Parameter(s):
CLOUD HEIGHTCLOUD VERTICAL DISTRIBUTION
Platform(s):
ICESAT
Sensor(s):
ALTIMETERS, GLAS
Data Format(s):
Binary
Temporal Coverage:
20 February 2003 to 11 October 2009
Temporal Resolution:
- 1288 minute
Spatial Resolution:
- 60 m to 70 m
- 60 m to 70 m
Spatial Coverage:
N:
86
S:
-86
E:
180
W:
-180
Blue outlined yellow areas on the map below indicate the spatial coverage for this data set.
Data Access & Tools
Documentation
User Guide
Product Specification Documents
Help Articles
How to Articles
Many NSIDC DAAC data sets can be accessed using the NSIDC DAAC's Data Access Tool. This tool provides the ability to search and filter data with spatial and temporal constraints using a map-based interface.Users have the option to