Throughout August, Arctic sea ice extent continued to track two or more standard deviations below the long-term average. The month saw two very strong storms enter the central Arctic Ocean from along the Siberian coast. In the Antarctic, ice extent remained near average.
Overview of conditions
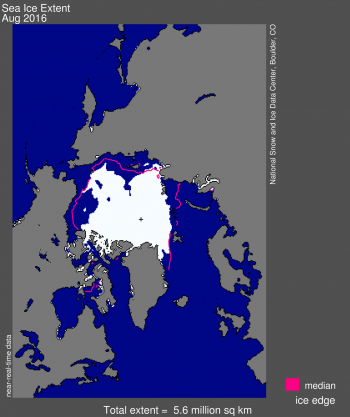
Figure 1. Arctic sea ice extent for August 2016 was 5.60 million square kilometers (2.16 million square miles). The magenta line shows the 1981 to 2010 median extent for that month. The black cross indicates the geographic North Pole. Sea Ice Index data. About the data
Credit: National Snow and Ice Data Center
High-resolution image
Average sea ice extent for August 2016 was 5.60 million square kilometers (2.16 million square miles), the fourth lowest August extent in the satellite record. This is 1.03 million square kilometers below the 1981 to 2010 average for the month and 890,000 square kilometers (344,000 square miles) above the record low for August set in 2012. As of September 5, sea ice extent remains below average everywhere except for a small area within the Laptev Sea. Ice extent is especially low in the Beaufort Sea and in the East Siberian Sea. With about two weeks of seasonal melt yet to go, it is unlikely that a new record low will be reached. However, since August 26, total sea ice extent is already lower than at the same time in 2007 and is currently tracking as the second lowest daily extent on record. In addition, during the first five days of September the ice cover has retreated an additional 288,000 square kilometers (111,000 square miles) as the tongue of sea ice in the Chukchi Sea has started to disintegrate.
Conditions in context
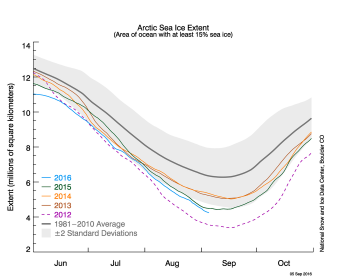
Figure 2a. The graph above shows Arctic sea ice extent as of September 5, 2016, along with daily ice extent data for four previous years. 2016 is shown in blue, 2015 in green, 2014 in orange, 2013 in brown, and 2012 in purple. The 1981 to 2010 average is in dark gray. The gray area around the average line shows the two standard deviation range of the data. Sea Ice Index data.
Credit: National Snow and Ice Data Center
High-resolution image
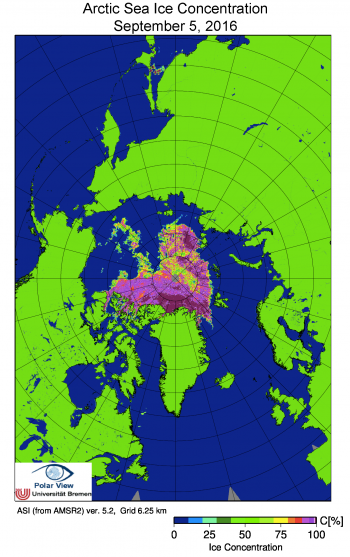
Figure 2b. The map shows Arctic sea ice concentration from the AMSR2 satellite instrument for September 5, 2016. Light blues and greens in ocean areas indicate areas of low ice concentration. The grey circle at the North Pole indicates where the satellite does not collect data, due to its orbit.
Credit: National Snow and Ice Data Center/University of Bremen
High-resolution image
The average ice loss rate through August was 75,000 square kilometers per day (29,000 square miles), compared to the long-term 1981 to 2010 average of 57,300 square kilometers per day (22,100 square miles per day), and a rate of 89,500 square kilometers per day for 2012 (34,500 square miles per day). Total ice extent loss in August was 2.34 million square kilometers (904,000 square miles).
Air temperatures at the 925 hPa level were 1 to 3 degrees Celsius (2 to 5 degrees Fahrenheit) below average for a large area stretching from the northern Kara Sea, through the Laptev Sea, and into north-central Eurasia. Temperatures elsewhere over the Arctic Ocean were near average. Reflecting the generally stormy pattern through the month, sea level pressures were well below average (as much as 10 hPa) over the central and eastern Arctic Ocean. Two very strong cyclones entered the central Arctic Ocean in August from along the Siberian coast, bringing strong winds. On August 16, the central pressure of the first cyclone dropped to 968 hPa, nearly rivaling the storm in early August 2012 that attained a minimum central pressure of 966 hPa. On 22 August, the second storm started moving to the central Arctic Ocean along a similar track, and on August 23, attained a central pressure of 970 hPa.
Past studies have shown that stormy summers tend to end up with more sea ice at the end of the melt season than summers with high pressure over the central Arctic Ocean, primarily because stormy summers are both fairly cool and the wind pattern tends to spread the ice out. However, the impact of strong individual storms may be different—the 2012 event appears to have temporarily boosted ice loss by breaking up the ice cover, with the wave action tending to mix warmer waters from below to hasten melt. It may also be that, as the ice cover thins, its response to storms is changing.
It indeed appears that the August 2016 storms helped to break up the ice and spread it out, contributing to the development of several large embayments and polynyas. Some of this ice divergence likely led to fragmented ice being transported into warmer ocean waters, hastening melt. Whether warmer waters from below were mixed upwards to hasten melt remains to be determined, but as discussed below, these storms were associated with very high wave heights.
August 2016 compared to previous years

Figure 3. Monthly August ice extent for 1979 to 2016 shows a decline of 10.4 percent per decade.
Credit: National Snow and Ice Data Center
High-resolution image
Arctic sea ice extent averaged for August 2016 was the fourth lowest in the satellite data record. Through 2016, the linear rate of decline for August is 10.4 percent per decade.
Cyclones, ocean wave heights, and ice retreat
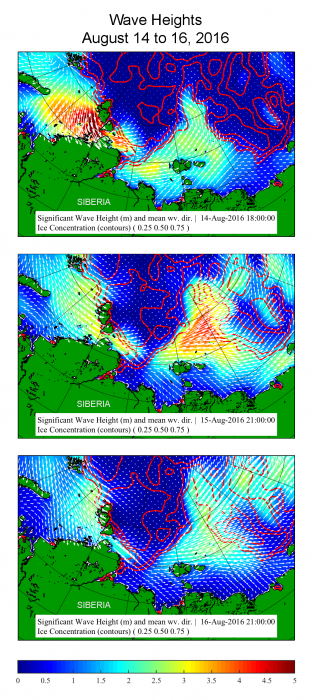
Figure 4. This series of plots shows significant wave height (in meters, indicated by color scale) in the western Arctic Ocean during the 2016 Arctic cyclone, from August 14 to August 16, 2016, as predicted by a numerical wave model (WAVEWATCH III), run at the Naval Research Laboratory (NRL). The solid red lines correspond to the analysis ice concentrations (25 percent, 50 percent and 75 percent) used as input for the wave model. White arrows indicate wave direction. This hindcast uses two time-varying inputs: 10-meter wind vectors from the atmospheric model NAVGEM (Navy Global Environmental Model, Hogan et al. 2014) run at the Fleet Numerical Meteorology and Oceanography Center (FNMOC), and analyses of ice concentrations (also produced at FNMOC) from passive microwave radiometer data (SSM/I). The wave model is run on a polar stereographic grid with a resolution of approximately 18 kilometers.
Credit: Erick Rogers, Naval Research Laboratory
High-resolution image
Large waves are common at high latitudes; 10-meter wave heights (33 feet) are not unusual for the Nordic Seas, and 15-meter wave heights (49 feet) can occur in the high latitudes of the Southern Ocean. However, large waves are a relatively new feature of the western Arctic Ocean. The height of waves is in part determined by surface wind speed, as well as the fetch (distance over open water that the wind can travel) and the duration of a wind event. A moderate sea ice cover damps ocean waves by absorbing and dispersing the wave energy through jostling of the ice floes against one another. A dense ice pack cover acts as a shield between the ocean and the surface wind, preventing wave formation.
In the latter half of the twentieth century, 4 to 6 meter waves (13 to 20 feet) rarely occurred in the western Arctic Ocean, but with more open water they have become more frequent, especially when strong storms enter the Arctic Ocean in late summer or early autumn. During the first of the two August cyclones discussed above, waves up to 5.9 meters (19 feet) were predicted. This occurred during the early part of the cyclone’s lifecycle (1800 UTC August 14), in the eastern Kara Sea. Further east, north of the New Siberian Islands, wave heights were estimated as high as 4.3 meters (14 feet) late on August 15. In this region, the waves were directly incident on the ice edge. In response, the ice edge retreated following the 4.3 meter waves on August 15.
Northwest Passage update
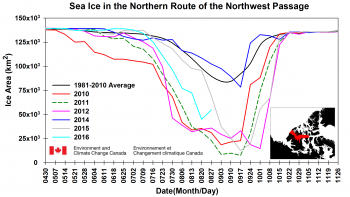
Figure 5. The time series shows total sea ice area for selected years and the 1981-2010 average within the northern route of the Northwest Passage. The cyan line shows 2016 and other colors show ice conditions in different years. Data are from the Canadian Ice Service.
Credit: Stephen Howell, Environment and Climate Change Canada
High-resolution image
The Northwest Passage refers to the fabled shortcut between the Atlantic and Pacific through the Canadian Archipelago. However, it is not one route. There is a northern, deep-water route through the Parry Channel, entered from the west through the M’Clure Strait and a shallower southern route, known as Amundsen’s route. Sea ice in the Parry Channel route has shown a sharp decline since the middle of July, but the channel is still not entirely ice free. Considerable ice remains in the western (M’Clure Strait) region and there are lesser amounts in the eastern regions. This is mostly (~80 percent) multiyear ice. Low ice years in the Parry Channel are typically the result of early summer breakup associated with high sea level pressure over the Beaufort Sea and Canada Basin that displace the Arctic Ocean pack ice away from the western entrance. Conversely, low sea level pressure anomalies over the Beaufort Sea and Canada Basin keep the Arctic Ocean pack ice up against the western entrance. This has been the case for much of the 2016 melt season. The southern (Amundsen’s) route is open but it is still uncertain whether the northern route will open in the coming weeks.
Even during mild ice years, thick multiyear ice is typically advected into these routes during the summer months. Multiyear ice is a significant obstacle for ships. Nevertheless, taking advantage of mild sea ice conditions, the 68,000-ton Crystal Serenity set sail from Anchorage, Alaska on August 16 for its 32-day journey through the Northwest Passage via Amundsen’s route. This is the largest ship thus far to navigate the Northwest Passage and is accompanied by an icebreaker ship and two helicopters. The ship sailed through the Northwest Passage in less than three weeks—52 times faster than Amundsen’s nearly three-year voyage.
On the other side of the Arctic, the Northern Sea Route appears mostly ice free.
Further reading
Collins, C. O., W. E. Rogers, A. Marchenko and A. V. Babanin. 2015. In situ measurements of an energetic wave event in the Arctic marginal ice zone. Geophysical Research Letters, 42, doi:10.1002/2015GL063063.
Haas, C., and S. E. L. Howell. 2015. Ice thickness in the Northwest Passage. Geophysical Research Letters, 42, 7673–7680, doi:10.1002/2015GL065704.
Hogan, T., et al. 2014. The Navy Global Environmental Model, Oceanography, 27(3), 116-125.
Howell, S. E. L., T. Wohlleben, M. Dabboor, C. Derksen, A. Komarov and L. Pizzolato. 2013. Recent changes in the exchange of sea ice between the Arctic Ocean and the Canadian Arctic Archipelago. Journal of Geophysical Research, 118, 3595–3607, doi:10.1002/jgrc.20265.
Thomson, J., and W. E. Rogers. 2014. Swell and sea in the emerging Arctic Ocean, Geophysical Research Letters 41, doi:10.1002/2014GL059983.
Thomson, J. et al. 2016. Emerging trends in the sea state of the Beaufort and Chukchi seas, Ocean Modelling 105, doi:10.1016/j.ocemod.2016.02.009.